Skipping off the Sky
From our backyards we propagate radio signals up towards the heavens. And they would keep going into outer space if it was not for layers of charged particles floating miles above us. These layers of charged particles are ionized clouds—concave formations wrapping around the planet. Our radio signals are refracted by these electric clouds, causing their trajectories to be redirected back towards the ground.
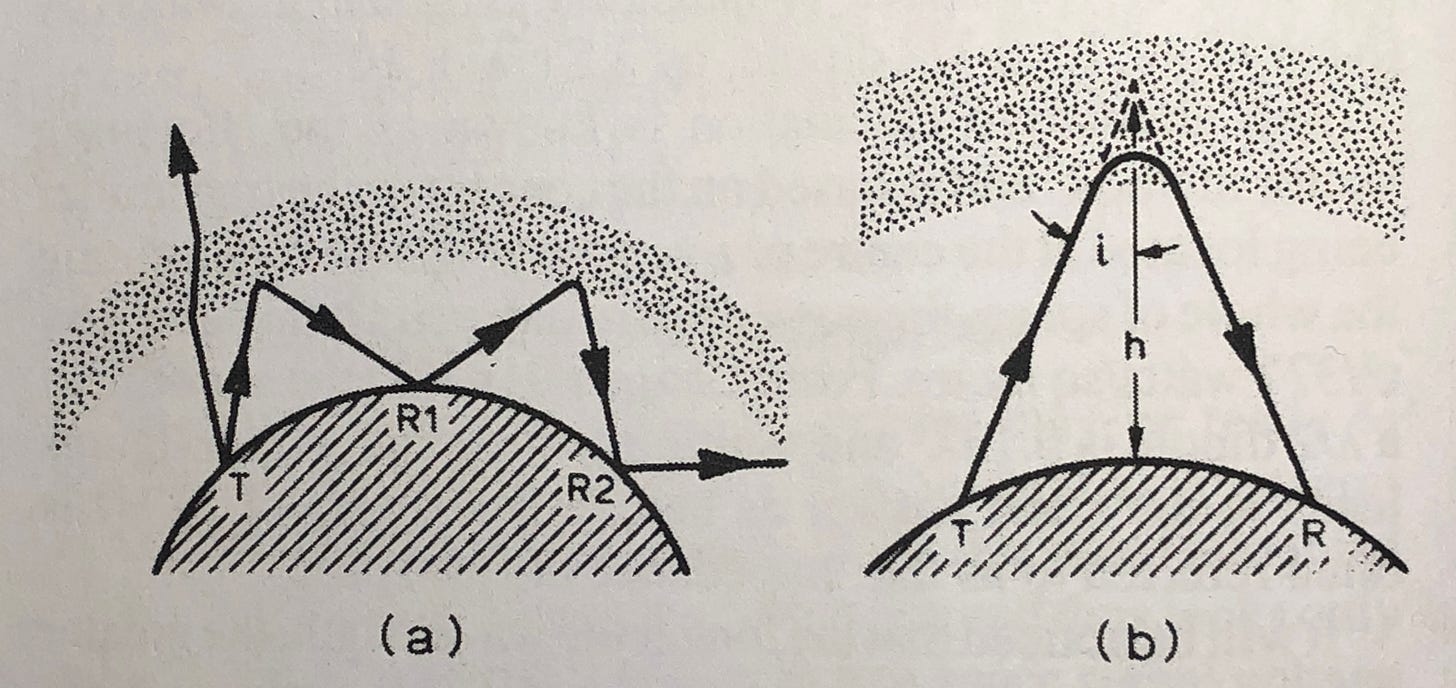
Ground Loss per Hop
Generally speaking, ground losses associated with ionospheric “hops” reduce our signal 50% (3 dB) per hop. Moreover, the longest terrestrial span via ionospheric hop is about 1,900 miles—with one exception discussed shortly. When put together, this means East Coast stations working into Europe have an advantage over Midwest stations, and about twice that over West Coast stations. Naturally, these generalizations are introduced for the sake of our discussion; your actual mileage may vary.1
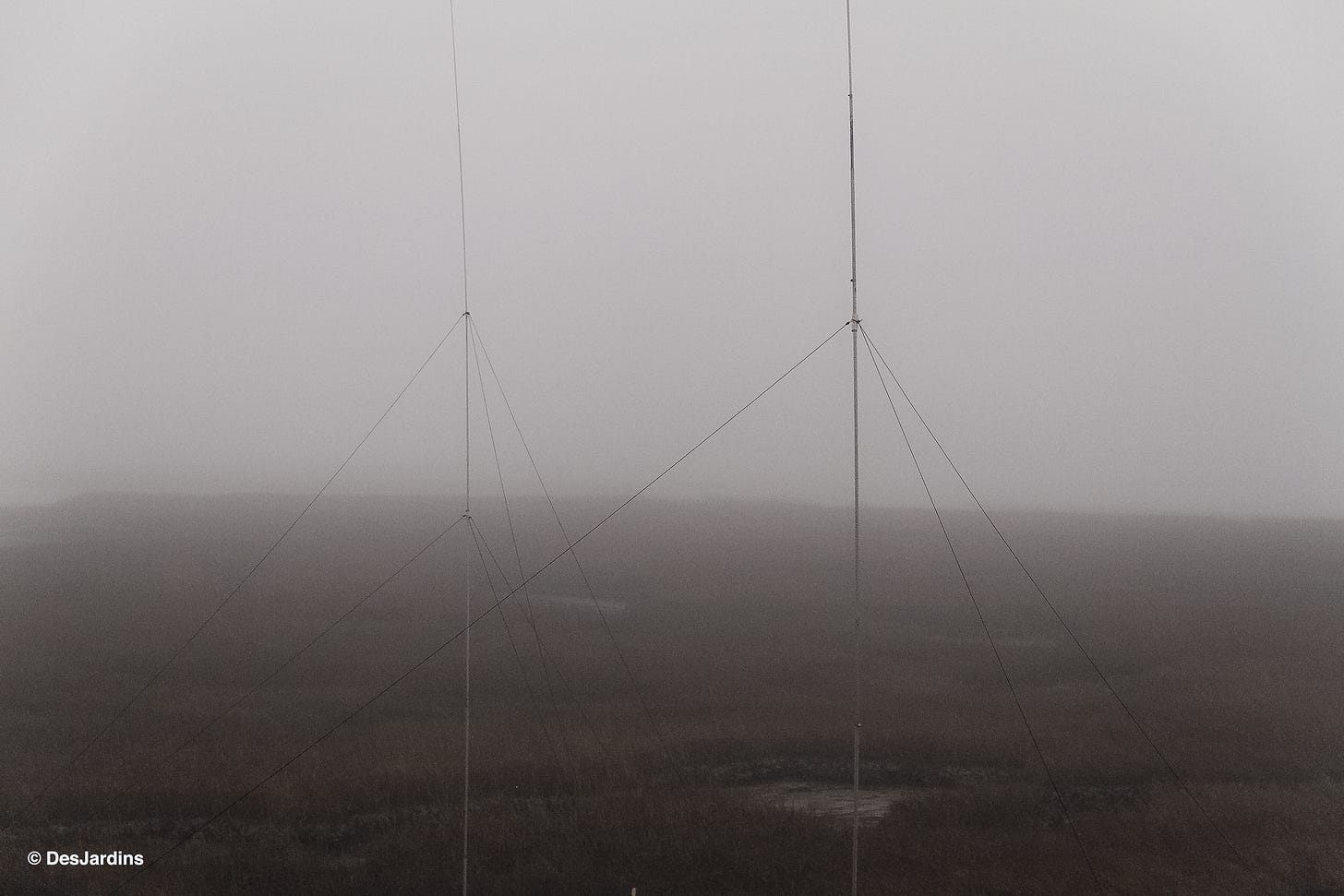
We mention all of this because the 1,900 mile limit per (F-layer) hop assumes the signal is emitted at the lowest possible elevation angle. If it’s not—which is usually the case—additional ionospheric hops are required to complete the 1,900 mile span. We don’t like that because each hop reduces the signal reports we get from other hams.
So how did low “take-off” angles get associated with radio signals propagating long distances (DX)? The analogy of skipping stones across a pond helps us understand.
Angle of Incidence = Angle of Reflection
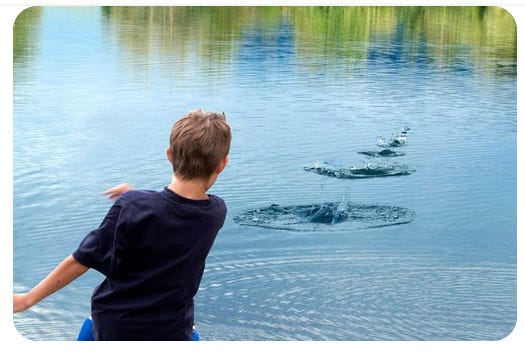
When we skip flat stones off a pond, we try to skip them as far as possible. To do that, we throw them so they encounter the pond’s surface at oblique (small) angles knowing the stones will ricochet off at the same shallow angles. The takeaway is that the angle of incidence equals the angle of reflection. In other words, the angle at which the stones hit the water equals the angle at which they fly off it.
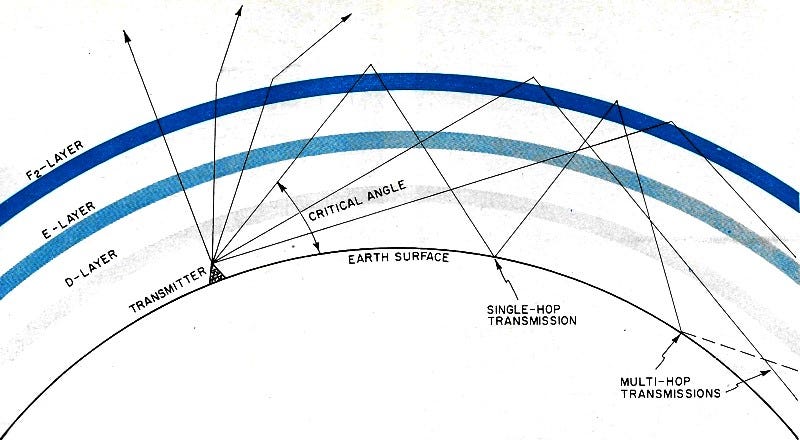
Inverted Pond Suspended Over Our Heads
Skipping stones off ponds testifies to what happens when radio energy is emitted up towards the sky. For the purposes of this discussion, we can envisage the ionosphere as an upside-down pond suspended hundreds of miles over our heads. When we send a radio wave up towards this firmament, the lower its trajectory angle the further it travels before skipping off the ionosphere. When we raise its trajectory angle, the wave encounters the ionosphere a lot earlier and at higher incidence angles than it did before. This reduces the distance it spans over the ground per each ionospheric hop. If we increase its angle of trajectory even further, the wave encounters the ionosphere almost perpendicular to it. And like a stone thrown directly down into the water, the radio wave passes through the ionosphere and continues into outer space. When this happens, we say that the trajectory angle of the radio wave is above the critical angle, as denoted in the diagram above.
Let Curvatures Do the Work
We hams try to communicate over great distances by emitting radio energy in the hope it will bounce off the ionosphere as few times as possible before arriving at another ham’s location (QTH). To do this we use antennas that emit radio energy at low elevation angles to shoot our signal out just above the horizon so as the ground falls away due to the curvature of the earth, our signal climbs evermore higher in the sky.
After propagating several hundred miles along this path unabated, it is the curvature of the ionosphere which causes our signal to slam into it, and to be refracted by it back down to the earth. The takeaway is the further a signal propagates before encountering the ionosphere, the greater the distance it spans across the earth’s surface. Hopefully it is now evident to readers that radio signals emitted at low elevation angles maximize the terrestrial distances spanned per ionospheric hop, and this is why long distance radio communication is best pursued with antennas emitting energy at low “takeoff” angles.
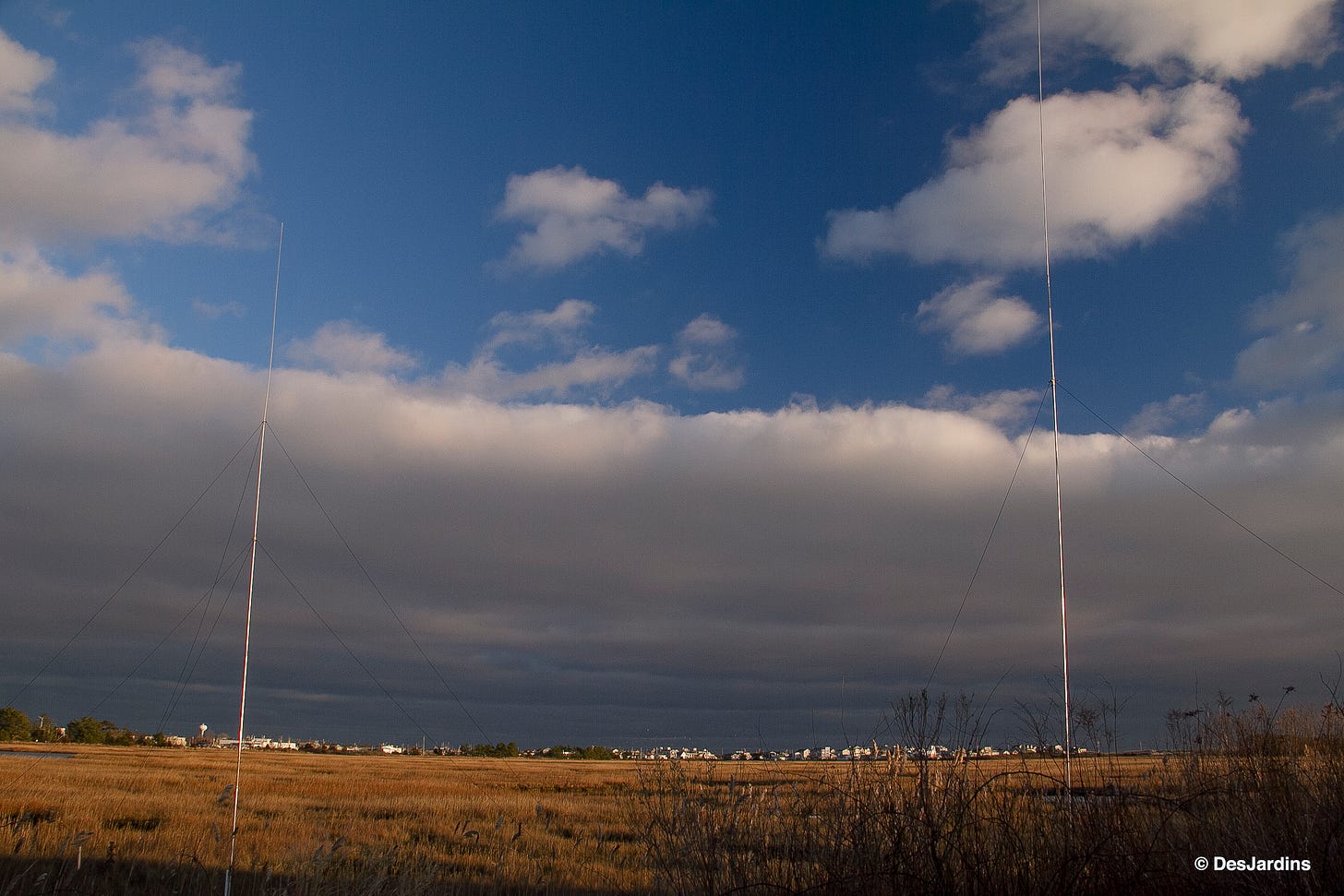
A brief word about the exception to the 1,900 mile maximum rule: chordal-hop propagation. This type of propagation takes place across the nightside of the earth, and is only accessible through a space-time portal. Catching chordal-hop propagation is like catching an overnight flight—you have to be at the boarding gate on time. And it only extends between radio operators on opposite sides (antipodal) of the planet when one is near dawn and the other is near dusk. As soon as either emerges into day or slips into night, the party’s over and they can’t stay there.
In a Nutshell
Essentially, what happens is that the first time your signal hits the ionosphere, it is not redirected back down to the earth. Instead, it repeatedly skips off the underside of the ionosphere throughout the nighttime region of the planet. Its last skip occurs where the ionosphere reemerges back into daylight. It is then, and only then, that your signal bounces back down to earth.
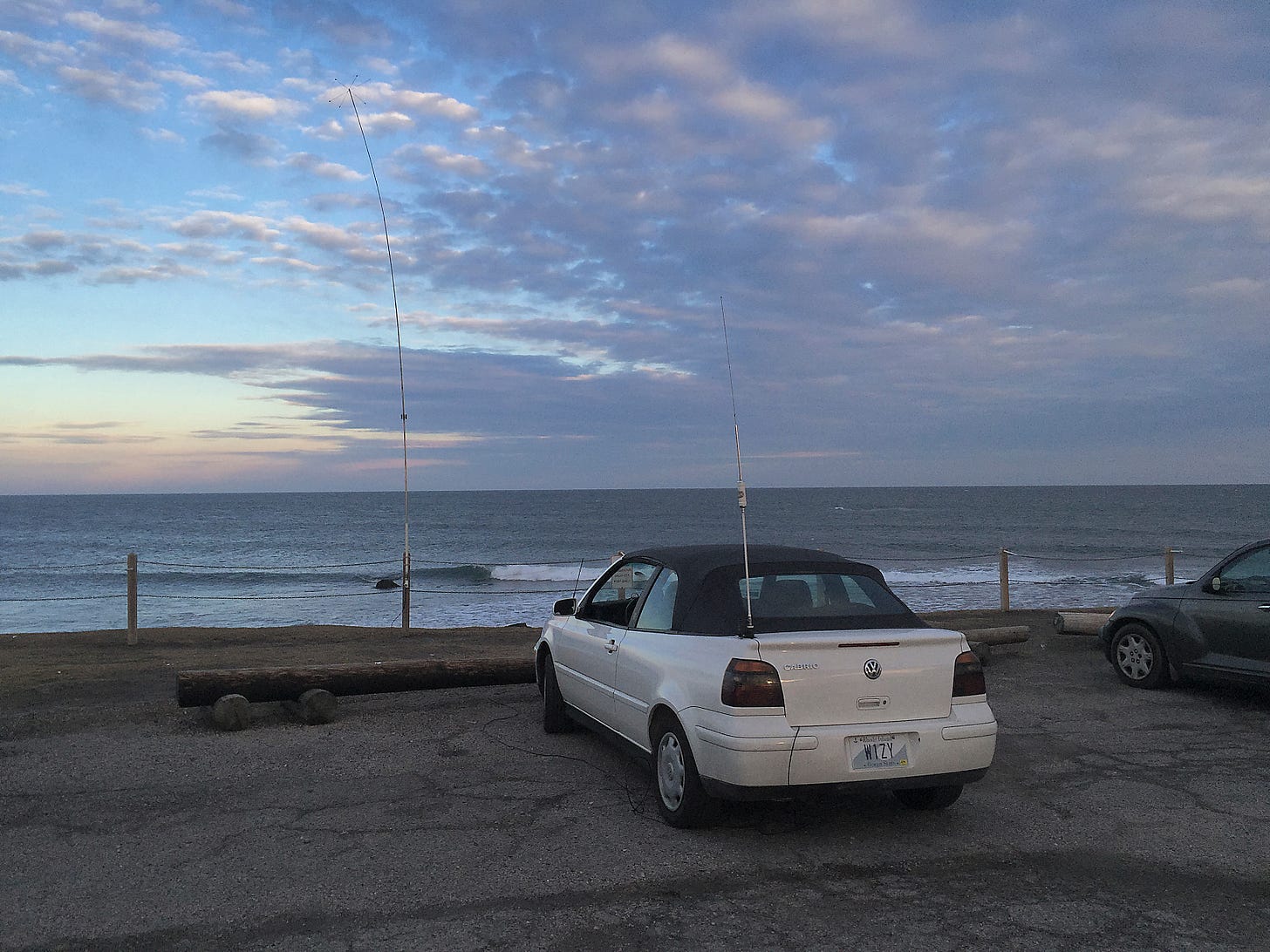
Thus, chordal-hop transports your radio signal halfway around the planet without any ground loss. Combined with the fact that you can only “get onboard” at dusk or dawn, chordal-hop opens exciting possibilities for portable seaside operations.
How It Works: Ionosphere Skewed Upwards at Night
On the daylight side of the earth the sun bombards gas molecules in the upper atmosphere, knocking electrons off which ionizes them. This ionization settles into several layers during the day. As the earth passes into night, the sun no longer shines so the free electrons recombine with their gas molecules. This dissipates the lower ionized layers while thinning out the uppermost (F) layer, which, in turn, raises its effective height above ground. That’s why you can hear AM radio stations really far away only at night.
If we were looking at the earth from outer space, we would see that the upper ionosphere is a lot closer to the daylight side than it is to the nighttime side. Within the dusk/dawn transition (terminator) between the two, we would note that the ionosphere slopes upwards as it enters into dusk and downwards as it emerges into dawn. This upward and downward tilting of the ionosphere within the earth’s terminator facilitates the entry and exit portals of chordal-hop propagation.
Varying Index of Refraction & Sloping Contour
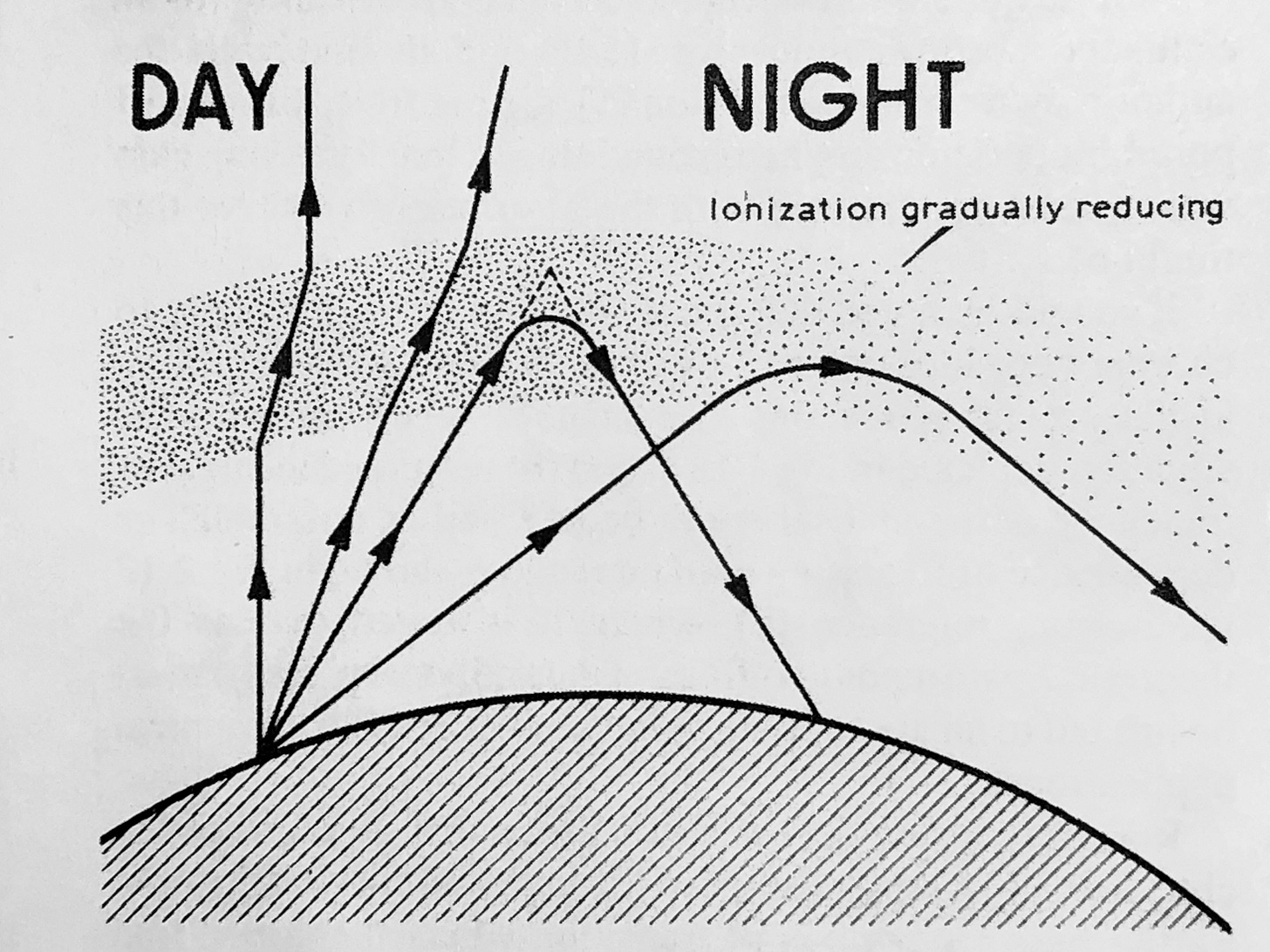
When your signal enters the ionosphere at the point where the sun has just dipped below its horizon, it passes through a region of gradually reducing ionization which gradually reduces the rate at which the signal bends (index of refraction) until it emerges at an angle so unusually shallow that it repeatedly skips off the underside of the ionosphere across the night side of the planet. The signal is not attenuated by ground reflections because it skips off charged particles. This is a beautiful thing.
AUDIO: Click to hear Japan & Korea long path in a car
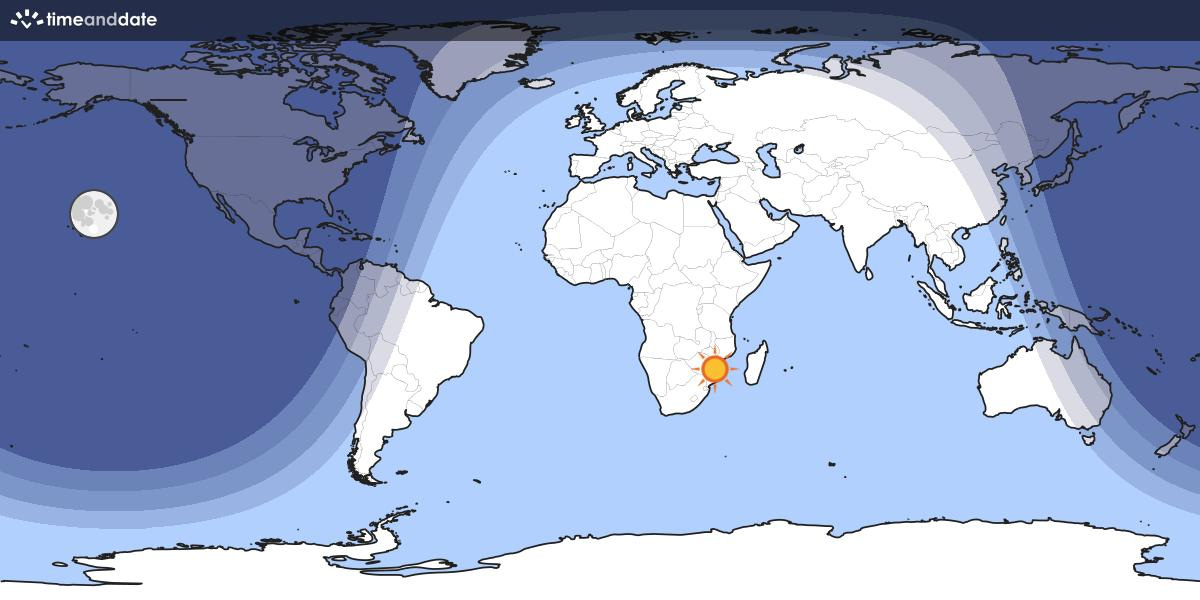
If you try chordal-hop at home one late afternoon, call out eastwards into the encroaching night, or, twelve hours later, listen for signals emerging from the night as it recedes to the West.
Audio: JA5CJZ at 59 +30dB on a 7 MHz mobile whip
Hearing chordal-hop signals requires knowing where the “grayline” terminator is located in realtime, and what antipodal regions are within range of chordal-hop portals. Click on the world map below to be taken to it in realtime.
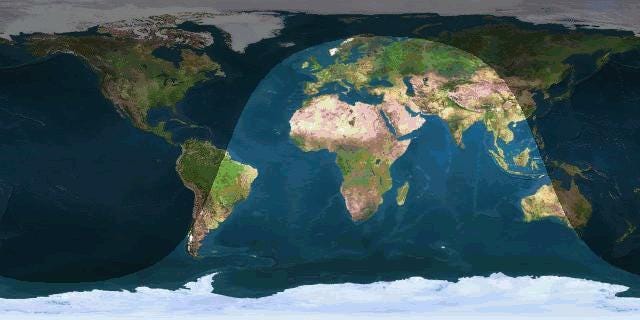
Check out Chapter 2, here.