Horizontal & Vertical Radiation Patterns
Our discussion about vertical antennas begins by examining the radiation pattern of a 1/2-wave horizontal dipole in free space, as depicted below.
We’ve all seen this image before. Its the radiation pattern of a 1/2 wavelength dipole in free space. The shape is a “torus”— a bagel, if you’re from Brooklyn. In the figure above, we understand that the dipole wire is horizontal from our perspective, and passes through the torus—like an axle passing through a truck tire.
If we were floating in outer space broadside to the dipole we would experience its maximum signal strength (antinode). Should we then float around to either end—where the dipole visually presents itself to us as a dot—its signal strength would drop off next to nothing because we are looking into one of its deep nulls (node).
Observations
The first takeaway is that horizontal antennas exhibit nulls off their ends. There’s no getting around this. The second takeaway is that maximum radiation occurs perpendicular to the wire. In other words, a horizontal dipole spews out energy in front, behind, above and below itself. Off either end there is—for the purposes of our discussion—no energy radiated at all.
Vertical Radiation Pattern
Let us now focus on the figure appearing below depicting the radiation pattern of a vertical dipole in free space.
Clearly, this is the first pattern rotated 90º so that the dipole now passes vertically through the toroid. This should be self-evident to readers. What may not appear self-evident is what this means in a practical sense. Firstly, it means the dipole’s two nulls are now pointing up at the sky and down at the ground. Secondly, it means a vertical emits maximum radiation at the horizon (0º). Finally, it shows that this low-elevation energy is equally dispersed in all compass headings—North, South, East and West.
Horizontal vs. Vertical
When we compare the two, we realize horizontal antennas spew half their emissions towards the ground and the other half towards the sky—most of this component splaying at elevation angles not optimal for long distance radio communication (DX). And all of this occurring broadside to the antenna. When we move to either end, the radiation drops off precipitously because we are now looking down one of its deep nulls.
The situation for verticals is somewhat different. Maximum radiation is spewed at extremely low elevation angles in all compass headings. As you progress upwards through elevation angles less optimal for DX, the radiation drops off to the point where nothing is emitted straight up at the sky (90º) since there’s a null at the top of the vertical’s pattern.
Angle is King
So which antenna is better equipped to communicate over long distances? The horizontal antenna emitting a small fraction of its energy at the low elevation angles optimum for DX, or the vertical emitting maximum energy 360º at the horizon?
Thus far we have looked at the radiation patterns of horizontal and vertical dipoles in free space. When we bring these patterns down to the surface of the earth, the ground becomes a major determinant.
Ground Proximity Sculpts Antenna Patterns
Whereas a horizontal dipole spews half its energy towards the ground, a vertical emits far less because of its deep, center null. The takeaway is that horizontal antennas emit a lot more downward energy than verticals do. This is important because as antennas are lowered to the ground, the energy they emit downwards bounces back up at them. This changes their pattern’s shape.
Verticals Over Salt Water Default to Their Free Space Pattern
Since a vertical emits less downward energy than a horizontal antenna, its pattern is less susceptible to ground reflections. In the elevation plot, below, observe how little the pattern of a monopole vertical changes as it is lowered from 0.625 to 0.25 wavelength over a perfectly conducting plane.
Upon arrival over salt water, the monopole vertical defaults to its free space pattern, as depicted below by the green trace.1
Whereas monopole verticals default to their free space pattern as they approach the ground, the patterns of horizontal antennas are contoured by their ground reflections.
Horizontal Patterns
Let’s examine the isotropic pattern of a horizontal dipole upon being lowered to 1/2-wavelength above the ground.
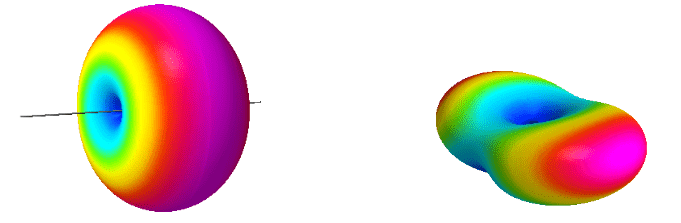
As things turn out, it morphs from a truck tire into an Idaho potato with a hole straight up through its center. How did that happen?
How Ground Reflections Sculpt Horizontal Patterns
As the horizontal isotropic pattern is brought closer to the ground, its downward energy is reflected back up at its above-ground energy. This causes the two energy envelopes to converge. At their points of convergence, the ground-reflected energy will have traveled further than the above-ground energy. This creates a phase difference between the two wavefronts.2
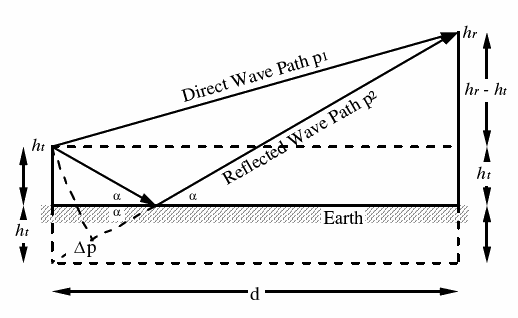
Where the two wavefronts converge in-phase, their electric fields “add up”—causing the isotropic pattern to protrude outward—forming a lobe. Where the two wavefronts converge out-of-phase, their electric fields “cancel out”—creating a null. At points between, wayward phase differentials sculpt the pattern’s shape between the lobes and the nulls.3
Sensing mathematics at play without understanding mathematics helps us understand how ground reflections sculpt the shape of horizontal antenna patterns.
You don’t have to be a physicist to recognize that complex mathematics is at play when the truck tire pattern is transformed into the potato pattern. If we look closely with our untrained eyes, we may be able to detect vestiges of the former in the latter.
Intuiting Mathematical Contours
I. Sides = Nulls
Whereas the truck tire pattern has two nulls lodged in opposing sides, the potato pattern is squeezed-in on the same two sides. This is no coincidence. The potato is squeezed-in on two sides because that’s where the isotropic side nulls used to be.4 Its oblong shape is a vestige of side nulls adorning the isotropic pattern that begot it.
The papa truck tire passed on its side null genes to the baby potato, which is why all horizontal antenna patterns are oblong in shape.
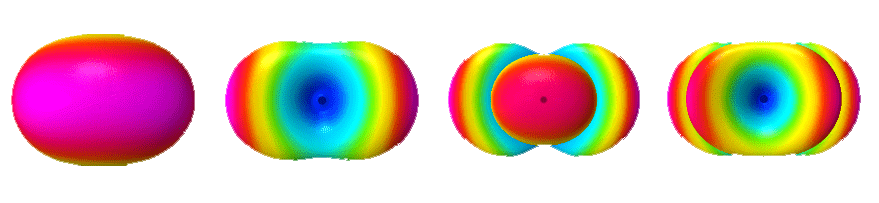
II. Broadside = Lobes
When broadside to a horizontal dipole, we observe maximum energy emitted in front of, behind, above and below the wire. Emissions directed towards us and away from us bounce off the ground and converge in-phase with the above-ground envelope forming forward and rear lobes. The range of elevation and azimuth angles over which the two wavefronts “add up” corral energy towards the lobes, producing the “hot spots” of electromagnetic emission colored magenta in the diagram, below.5
III. Center = Null
We must turn our attention to energy spewed sharply downwards when contemplating the origin of the potato pattern’s mysterious central null.6 Energy emitted downwards bounces back up and converges out-of-phase with energy being emitted straight up—creating the deep null in the middle of the potato pattern. Variations between the ground reflection and upward emission angles contour the interior of the null.7
Evolutionary Progression
Everything we have thus far discussed is based on a horizontal dipole suspended 1/2-wavelength over the ground. We’ve learned that this height institutes phase differentials between converging wavefronts shaping the pattern. Varying the suspension height varies the pattern, as seen below.
A cursory examination of these patterns reveals each one morphs into the other as the dipole is raised higher in the air. Without becoming pedantic and bogged down in details, it appears that every 1/4-wavelength increase in height constitutes an evolutionary step in the pattern’s contiguous development.
One Potato, Two Potato, Three Potato, Four!
Putting it colloquially so all readers might understand, at 1/4-wavelength above ground, the horizontal dipole’s pattern resembles an engorged tick. At 1/2 wavelength, the tick bifurcates (splits in two) upon the appearance of a central null—forming the potato pattern we’ve been studying. At 3/4 wavelength above the ground, the null morphs back into a tick seated atop the potato pattern. At 1 wavelength high, this second tick bifurcates upon the reemergence of the central null.
Ad Infinitum
Beyond 1 wavelength over the ground, we might expect to see this evolutionary pattern repeat itself: the tick reappears on top of the potato stack and then bifurcates into a potato upon the return of the central null. This appears to be exactly what’s going on, as suggested below. Click the image to enlarge it.

Eye Can See Clearly Now
The mathematical complexities of phase differentials are manifested in this progression, allowing us to conclude that ground proximity bifurcates horizontal antenna elevation patterns into lobes interspersed between nulls.
The Problem with Horizontal Antennas
“We need to pay particular attention to nulls, because multiple deep nulls are really what prevents us from hearing and working what are otherwise spectacular signals.”
—Tom, W8JI
Compare the elevation plot, above, with the elevation plot, below, depicting a 1/4-wave vertical at ground level over salt water.
The vertical’s elevation plot is distinguished by its lack of nulls.8 This means verticals are not susceptible to rapid fading characteristic of horizontal antennas whenever the propagation wave angle parks itself in a null. Under such conditions, slight variations of the wave angle cause an incoming signal to transit up and down the wall of the elevation null resulting in rapid fading of the signal’s reception.
“If the signal arrival varied just 1-2 degrees, (the) signal level could go from excellent readability to unreadable.”
—Tom, W8JI
The same holds true on transmit. For example, the audio clip below depicts a British station reporting a horizontal yagi instantaneously dropping into the noise in the middle of a shootout with a pair of saltwater verticals.
Deep Dive into Nulls
Just as there is nothing that be done about a horizontal antenna emitting little energy off its ends, there is little that can be done about the elevation nulls in its “vertical beamwidth”—aside from stacking several of them on top of and aside each other, as W8JI postulates below.9
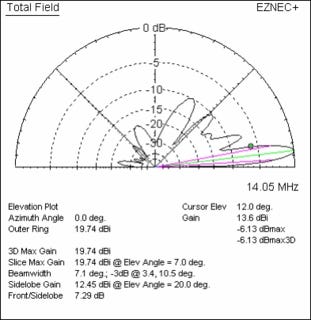
Tom Rauch has written an excellent article (“The Myth of Take Off Angle”) about elevation nulls in horizontal antennas available here for interested readers. In the article, he plots a monopole vertical over land and concludes its pattern is so similar to that of a horizontal dipole that any distinction between the two is not worth mentioning. He then moves on talking exclusively about horizontal antennas. Thus, once again, verticals are dismissed by yet another landlocked Midwesterner.
Vertical Lives Matter
However, out here on the East Coast we can provide verticals with the fair playing field afforded by salt water, allowing them to operate in their purest form. And when we do, they not only radiate below the 2x2 H-stack, but do so without any elevation nulls—from the pseudo-Brewster angle all the way up to the top.
This puts saltwater verticals into a class of their own, thus raising the question of how to push them to their limits. Additional “shootouts” with high horizontal antennas does not appear too promising. Perhaps the best way is to pit saltwater verticals against nature, itself, by testing their very low elevation emissions. We cover this in Chapter 3.
The other traces are for inland installations over different qualities of soil. The reader can see from these traces that the lower elevation energy of the monopole vertical is eradicated—obviously by the ground. This is discussed later on in the chapter.
Both wavefronts are oscillating at the exact same frequency because they are emitted by the same source. So when one travels farther than the other, they are no longer synchronous, in terms of their phase. At some distances they are in-phase—this forms a lobe. At other distances they are 180º out-of-phase—this forms a null. At distances between these points varying phase differentials contour the pattern’s shape between the in-phase lobes and the out-of-phase nulls.
This occurs in three spatial dimensions.
Since there is no energy emitted off the ends of a horizontal dipole in free space—for the purposes of our discussion—there is little hope to see anything to occur off either of its sides when the dipole is lowered to the ground. This is exactly what we see happen.
Readers may recognize this as one way “gain” is gained by a dipole. Sorry about that! 8D
The formation of forward and rear lobes occurs further out in the aerial’s far field than do convergences between energy reflected off the ground directly below the radiator and upward components. These convergences occur within the radiator’s reactive near field, radically contouring the center of its pattern into nulls and lobes every 1/4-wavelength increase in the radiator’s height above the ground.
Through their corresponding phase differentials.
Except for the one at the top.
This is very heavy-duty!